Nuclear physics is devoted to the understanding of the basic properties of atomic nuclei and the interactions involving hadrons and nuclei. While the study of such interactions can be performed in a very reliable way for light nuclear systems, the complexity of calculations increases with the number of particles involved. In this case, sophisticated many-body techniques have been developed to produce accurate results using as few approximations as possible.
The outcome of many years of high-quality research has fructified in a very good phenomenological description of nuclear structure, masses and sizes, as well as in the formulation of realistic baryon-baryon potentials that are used to describe compact astrophysical objects, such as neutron stars. Despite all this progress, a precise understanding on how nuclear bulk properties and hadronic interactions emerge from the fundamental theory of the strong interaction, quantum chromodynamics (QCD), is still lacking. The large complexity of the quark-gluon dynamics prevents the analytic solution of QCD in the energy regime relevant for nuclear physics, allowing only numerical solutions obtained through large-scale computations. Alternatively, the formulation of interaction Lagrangians in terms of effective hadronic degrees of freedom has proven to be an efficient way to describe a variety of low-energy nuclear phenomena.
Ultracold Atomic Physics has nowadays evolved into a very rich research field with several branches which cover from basic quantum many-body theory to Bose-Einstein condensation and related phenomena, and also with overlaps with condensed matter theory. Since the experimental achievement of Bose-Einstein condensation in the late 90s, the field has greatly broadened its scope. Right now, the experimental realization of ultracold atomic gases trapped in different geometries, optical lattices, etc., allows one to study the onset of quantum correlations in a large variety of settings, for instance, in spinor gases, dipolar gases, small Bose-Hubbard systems or binary mixtures. Recently, also the effects of dimensionality are being explored both theoretically and experimentally in few-atom systems.
OUR CONTRIBUTION
The research performed by nuclear physicists at ICCUB has far-reaching implications, playing an important role in the study of heavy ion collisions and in the equation of state of neutron-rich matter, and involving a wide range of phenomena, from the physics of matter at the femtoscale to the structure and dynamics of neutron stars.
In addition, the group has been successful in performing the numerical calculations required to make the connection with QCD, thanks, in part, to the ability to secure Tier-0 computational resources at international infrastructures.
Regarding to the contribution on atomic physics, the ICCUB researchers have been involved in recent years in the study of Bosonic Josephson junctions, vortex dynamics, spinor dynamics, soliton dynamics in condensates, synthetic spin-orbit coupling, and artificial gauge fields.
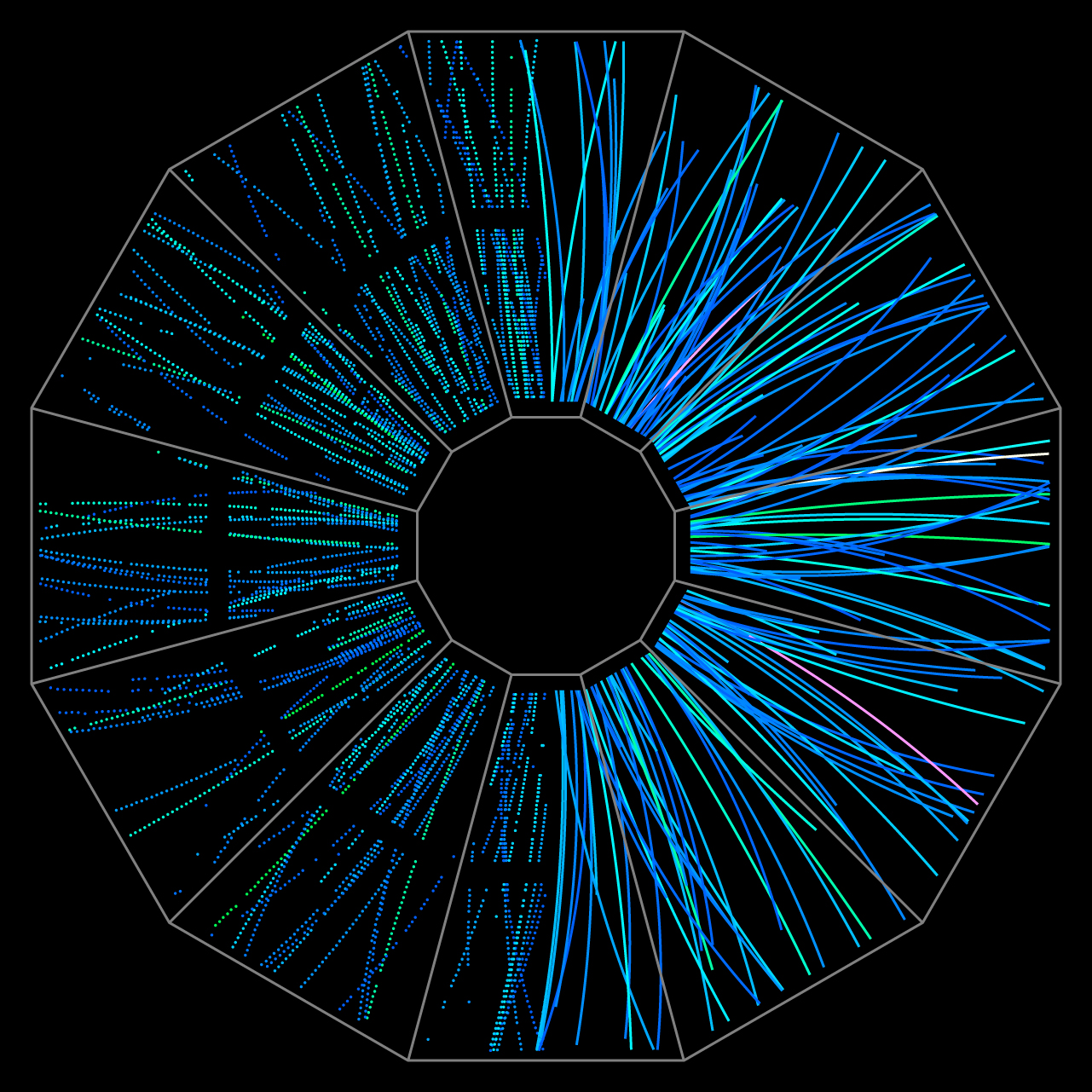
LINES OF RESEARCH
- Hadronic physics. Strangeness and charm in the nuclear medium
- Lattice QCD calculations of light nuclear and hypernuclear systems
- Nuclear structure. Nuclear symmetry energy.
- Relativistic heavy ion collisions
- Dense and hot nuclear matter and applications in nuclear astrophysics
- Radiation transport and interactions of radiation with matter
- Ultracold atomic physics and quantum many-body correlations
MORE INFORMATION